Quantum computing, with its promise of revolutionizing various industries through unprecedented computational power, stands at the frontier of modern technology. However, despite the theoretical advancements and impressive experimental demonstrations, there are significant challenges that must be overcome to make quantum computing practically viable and accessible in real-world scenarios. This article delves into the core challenges in practical quantum computing and the hurdles to its real-world accessibility.
1. Quantum Decoherence and Noise
Decoherence is one of the most critical issues in quantum computing. Quantum bits, or qubits, rely on maintaining a delicate state of superposition and entanglement to perform calculations. However, these states are extremely sensitive to external disturbances such as electromagnetic noise, temperature fluctuations, and even cosmic rays.
Noise and decoherence cause qubits to lose their quantum properties, leading to errors in computation. The time a qubit can maintain its state, known as coherence time, is currently very short. Developing qubits that can maintain coherence longer is a significant area of research. Approaches like error correction codes and quantum fault tolerance are being explored, but these solutions require additional qubits and complex algorithms, making the system more complicated and resource-intensive.
2. Error Rates and Quantum Error Correction
Quantum error correction (QEC) is essential for practical quantum computing, given the high error rates associated with quantum operations. Unlike classical bits, which are either 0 or 1, qubits can exist in multiple states simultaneously, and any interaction with the external environment can lead to errors. QEC involves encoding quantum information in a way that allows the detection and correction of errors without measuring the quantum information directly.
However, QEC is extremely resource-intensive. For every logical qubit (the qubit that performs actual computation), several physical qubits (the qubits that support error correction) are required. This overhead significantly increases the number of qubits needed, making it challenging to scale up quantum computers to a level where they can solve practical problems.
3. Scalability of Quantum Systems
Building a quantum computer that scales to hundreds, thousands, or millions of qubits while maintaining low error rates is a monumental challenge. Current quantum systems, such as those using superconducting qubits or trapped ions, face difficulties in maintaining coherence and connectivity as the number of qubits increases.
Superconducting qubits, for instance, require extremely low temperatures (near absolute zero) to function, necessitating complex and expensive cooling systems. Trapped ion systems, on the other hand, require precise control of ions with lasers, which becomes increasingly difficult as the number of ions grows. Researchers are exploring various architectures and technologies, but finding a scalable, stable, and cost-effective solution remains a significant hurdle.
4. Complexity of Quantum Algorithms
While quantum algorithms like Shor’s algorithm for factoring large numbers and Grover’s algorithm for database search have shown theoretical promise, developing new quantum algorithms for real-world applications is complex. Quantum algorithms require a deep understanding of both quantum mechanics and the specific problem domain, which limits the number of researchers who can contribute to this field.
Moreover, many quantum algorithms provide speedup only for certain types of problems. Identifying problems that can benefit from quantum speedup and developing corresponding algorithms is an ongoing challenge. Additionally, implementing these algorithms on current noisy intermediate-scale quantum (NISQ) devices requires further research and optimization.
5. Integration with Classical Systems
For quantum computers to be practically useful, they need to integrate seamlessly with existing classical computing infrastructure. Hybrid quantum-classical algorithms, where quantum computers handle specific parts of a problem and classical computers manage the rest, are seen as a practical approach. However, achieving efficient integration poses several challenges.
Data transfer between quantum and classical systems can introduce latency and errors. Moreover, developing software and frameworks that can leverage both quantum and classical resources efficiently is complex. Initiatives like the development of quantum programming languages (e.g., Qiskit, Cirq) and hybrid computing platforms are steps in the right direction, but more work is needed to create robust, user-friendly solutions.
6. Economic and Logistical Barriers
Building and maintaining quantum computers is currently very expensive. The infrastructure needed for quantum computing, such as cryogenic systems for superconducting qubits or vacuum systems for trapped ions, involves substantial costs. Additionally, the development of quantum technologies requires significant investment in research and development, skilled personnel, and specialized facilities.
These economic barriers make it difficult for many organizations, especially smaller companies and academic institutions, to access quantum computing resources. Cloud-based quantum computing services offered by companies like IBM, Google, and Amazon are helping to democratize access to quantum technology, but the high costs and limited availability of quantum resources remain significant barriers.
7. Standardization and Benchmarking
As the field of quantum computing evolves, there is a growing need for standardization and benchmarking to assess and compare the performance of different quantum systems and algorithms. Currently, there is no universally accepted standard for measuring quantum computational power. Metrics such as qubit count, coherence time, gate fidelity, and quantum volume provide some insights but can be difficult to compare across different technologies and architectures.
Standardized benchmarks would help in evaluating the progress and capabilities of various quantum technologies, guiding both researchers and potential users. Establishing such standards is a collaborative effort that requires input from academia, industry, and government organizations.
8. Education and Workforce Development
The specialized knowledge required for quantum computing spans quantum mechanics, computer science, and engineering, creating a high barrier to entry for new researchers and practitioners. Developing a skilled workforce is essential for advancing quantum technology and ensuring its practical implementation.
Educational institutions are beginning to offer courses and degrees in quantum computing, but there is a need for more comprehensive and interdisciplinary programs. Collaborations between academia and industry can help bridge the gap, providing students with hands-on experience and exposure to real-world applications of quantum computing.
Conclusion
While the promise of quantum computing is immense, realizing its potential in practical and accessible ways involves overcoming significant challenges. From technical hurdles like decoherence and error correction to economic and logistical barriers, the path to widespread adoption of quantum computing is complex and multifaceted.
Continued research and development, along with collaborative efforts across disciplines and industries, will be crucial in addressing these challenges. As technology advances and solutions emerge, the dream of practical, real-world quantum computing may become a reality, unlocking new possibilities and transforming industries in ways we are only beginning to imagine.
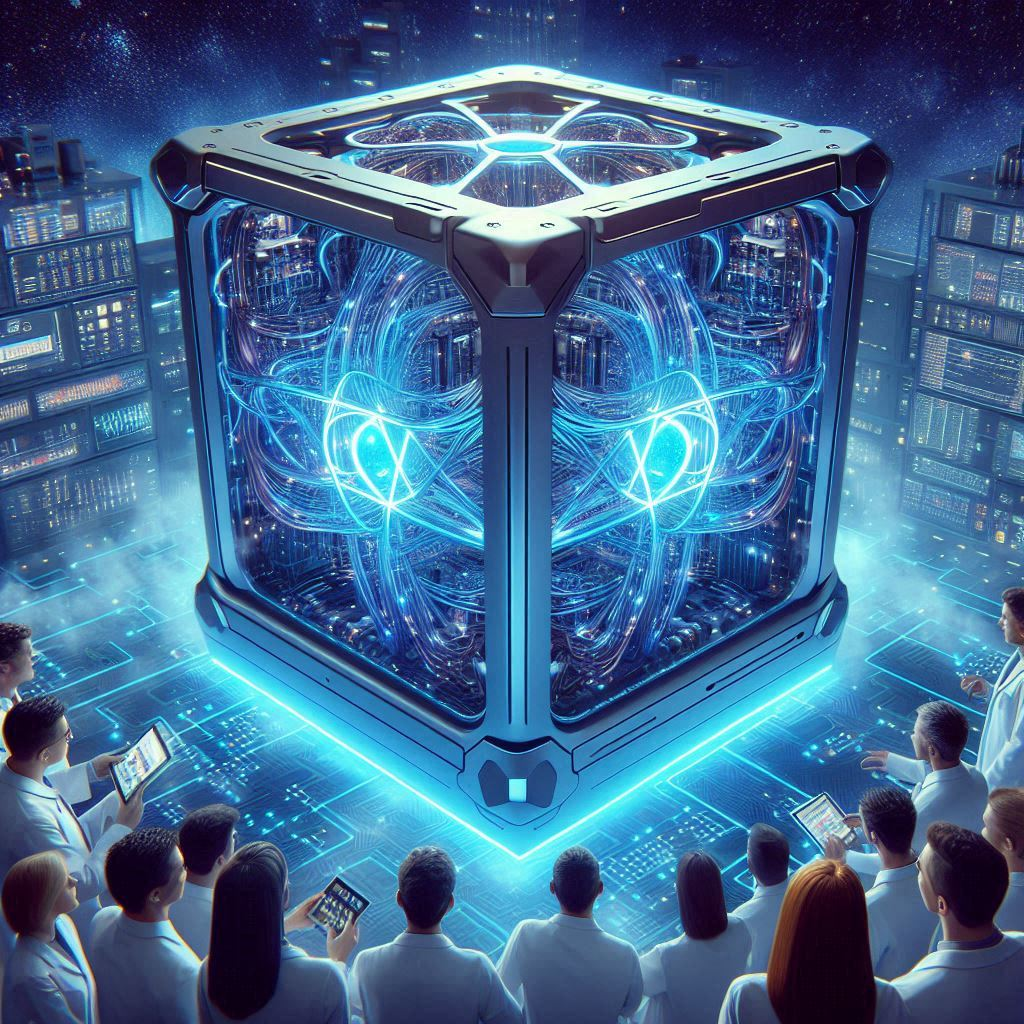